Mystifyingly, a particle beam is a stream of charged particles, including electrons and protons, often accelerated to almost the brink of light-speed.
It might be surprising, but the beams of particles created by accelerators like the Large Hadron Collider (LHC) at CERN are not only used to explore the spine-tingling fundamental forces of nature. They are also used in a range of boo-tiful applications including sterilisation of medical equipment and food products, mass spectroscopy and semiconductor fabrication for computer chips, as well as in medical imaging and cancer treatment applications, such as hadron beam cancer therapy (a new form of radiation therapy that uses protons and other ions).
These applications require precise control of the size and intensity of the beam. However, measurement of the beam to ensure it meets the specification required by these applications is problematic because either the beam is highly energetic and gruesomely destructive, or the particles are very rare and mischievously difficult to detect. Additionally, in medical applications there may be little time for optimisation between patients.
This demand has spurred the need for spook-takular methods to continuously assess the beam’s profile and quality in real-time without disrupting the beam itself.
As we learned in the article about magnetic fields the beam particles travelling inside particle accelerator are controlled using bending and focusing magnetic fields. To accommodate the acceleration of particles, it’s essential to scale up the magnetic fields in proportion to their momentum. To achieve dynamic adjustments in magnetic field strength, constant awareness of the beam’s properties and continuous parameter monitoring is crucial, all while preserving the beam’s undisturbed motion.
Traditional methods for monitoring beam characteristics use physical structures, such as intercepting foils which measure interactions, and scintillators that, when struck by an incoming particle, give off light from the energy they have absorbed.
However, such methods are not suitable for measurements in the LHC However, such methods are not suitable for measurements in the LHC as the potent beam possesses the capability to effortlessly go through these monitoring devices!
Researchers at the Cockcroft Institute have been developing a spookalicious gas screen as an alternative monitoring method.
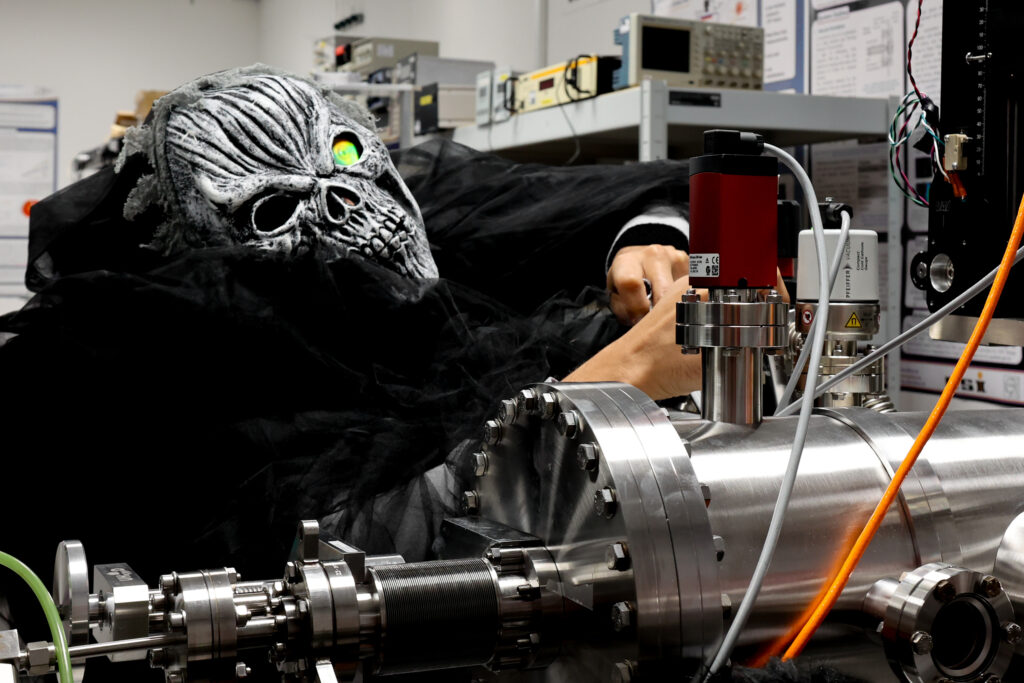
Supersonic expansion is used to create the fast, but cold gas curtain (animation: gas-jet shaping) as shown in the image below. The gas moves through a small opening from a region of high pressure into a region of very low pressure and expands faster than the speed of sound. There, it crosses the primary beam that needs to be analysed, and this collision produces light which is captured by a camera outside of the vacuum chamber.
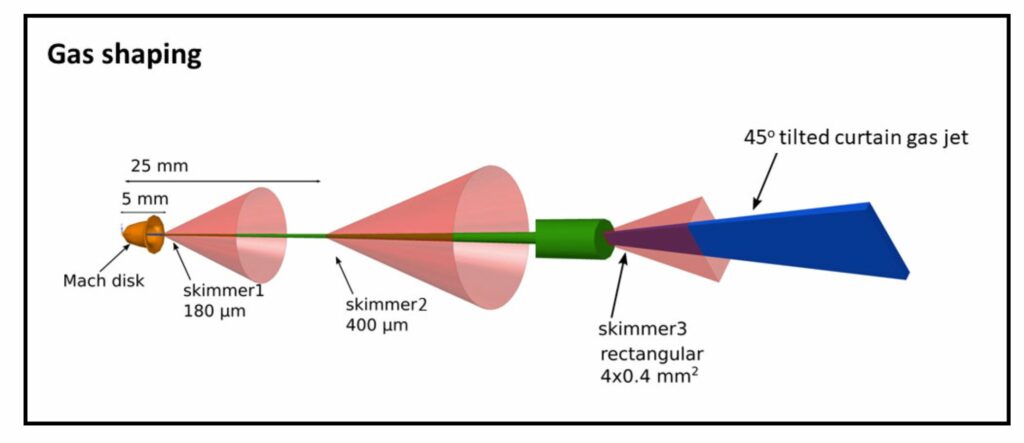
The captured image gives detailed information about the beam position and profile, its direction of travel, and intensity without disturbing the particle beam – a new technology that could be used in essentially any accelerator or light source.